How to use viruses to fight dangerous disease
Viruses have been detrimental to humans due to the nature of the diseases they cause. However, now, modified viruses can help in curing dangerous illness.
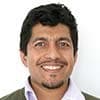
Viral Vector Senior Scientist
Since the dawn of time, viruses have been very detrimental to humans due to the nature of the diseases they cause. However, now, thanks to the advances in science, modified viruses can help in preventing and curing dangerous illness. Here one of our experts, Sam Stephen, takes us through his insights on how viruses are a key part of the fight against disease.
Viruses
We have concrete evidence that viruses have caused human diseases from as early as 1400 BC, as the hieroglyphs of Ancient Egypt show; from the humble flu to the devastating smallpox, viruses can decimate populations.
Viruses are microscopic superparasites. They tend to have very little means to survive by themselves, instead rely on their host to provide the main means of survival; some, like very bad guests who trash up their residence, destroy their host cells as they try to find more hapless hosts; others, like lentiviruses, ‘slip’ out of the hosts and keep them alive to produce more viruses. Typically, a virus represents the epitome in genetic efficiency. They have a very small genome with trans acting genes coding for multiple proteins sandwiched between cis-acting sequences essential for genetic replication and packaging. This genome is typically enclosed inside a protein shell (the capsid) along with few proteins essential to help the virus in its initial stages of infection, and the whole unit might be enclosed by an envelope to further protect and refine the selection of the host.
We recently celebrated the signing of peace at the end of WWI in 1918 in which over 20 million people were killed. But we tend to forget that in the very same year, a further minimum of 50 million people perished: not at the hand of their fellow humans, but in the hands of the simple influenza virus. This scale demonstrates the potential damage that viruses can cause. But can we harness their power for the benefit of humanity? This challenging research area has many opportunities for companies to find innovative solutions, which is precisely why CPI’s National Biologics Manufacturing Centre was founded.
There are two broad fields in which viruses can actually help us banish diseases-Vaccines and gene therapy.
Viral vaccines
Vaccines help us to prevent getting the disease in the first place; prevention is always better than cure. In 1796, on noticing that milk maids, who had been infected with a mild disease of cow pox, caused by the cow pox virus, which is a weaker counterpart of it’s more infamous cousin small pox, were immune to the deadly small pox. A rural doctor, Edward Jenner came up with the idea of exposing patients to cow pox to protect them from small pox. His initial cohort of test subjects included his own 11-month old son. Despite all the opposition, Jenner had developed the first modern vaccine (from the Latin word for cow, vacca), which went on to save millions of lives, if not billions. Louis Pasteur’s development of a rabies virus which lost its virulence, as a vaccine against the disease, opened up the field to help prevent the infection by dangerous viruses which did not have similar weaker viruses like the ‘cow pox-small pox’ duo.
Making use of the scientific knowledge available now, vaccines have come a long way since the time of Dr. Jenner. There are various approaches: inactivated (the virus is “killed”), live attenuated (the virus is propagated in a different condition to those in the human body: quite often, they are maintained at much lower temperatures), sub-particle vaccines (the virus is not just “killed”, it is broken up into bits and some of the protein bits are purified and presented as the vaccine), virus-like-particle (VLP) vaccines (the viral genome is absent, only the viral protein shell is present) and finally viral vectored vaccines (parts of the dangerous virus are presented by a benign virus to the human body). Each of these approaches have various pros and cons and at CPI, we have established a class-leading system to develop these vaccines to help others bring these out to the public.
Gene therapy
Gene therapy is another field in which viruses can help us to improve people’s health. Existing therapies usually focus on treating disease symptoms and not the cause. Genetic medicine involves any treatment that uses genetic units (DNA/RNA) to treat diseases themselves and not the symptoms. These advanced gene therapies that precisely target a specific cause of a disease are growing in popularity. To deliver this genetic medicine correctly, there is a need for a delivery mechanism (vectors) that are reliable enough for therapeutic applications. A non-viral way of delivering a piece of genetic medicine into the body is via a plasmid: a simple loop of DNA. To deliver a plasmid, physical methods can be used such as injections, coating the DNA with lipids or making the cells porous with electricity. Although plasmids do not require a vector to get into cells, the chance of the gene ending up in the right place is rare.
However, viruses are very good at targeting cells. Viruses naturally have all the tools they need to get into a cell and replicate itself. The genome of a virus can be divided into the absolutely essential part needed on the genome (cis-acting elements) and the parts which can be provided by other genomes (trans-acting elements). In gene therapy, we replace all these dangerous trans-acting viral genes with therapeutic ones, thereby greatly increasing the efficiency of delivering therapeutic DNA into a cell. A good analogy to describe the difference is that non-viral gene delivery methods are similar to old WWI era bombs which were dropped by pilots flying over the trench where the pilot had very little control over the direction in which the bomb would end up, whereas viruses are like the modern homing cruise missiles that can hit a fly sitting in the middle of a football field thousands of miles away.
In addition, viruses could be ideal vectors for delivering gene therapies since they have already got the methods to bypass the host immune system.
When selecting a gene therapy vector, there are lots of viruses to choose from. However, there are a few important viral characteristics to consider: diseases caused by the unmodified virus, ease of manipulation, the natural life cycle, cancer-causing potential and the expected yield for commercial manufacture. As a rule, these viruses cannot divide under normal conditions, though there are exceptions
The most common viruses currently used in the field are from three families: retroviruses (including lentiviruses) that have RNA as their genetic material, adenoviruses that have double stranded DNA for their genetic material and the adeno-associated viruses, which have single stranded-double stranded combo DNA genomes.
Gene therapy got its first taste of success in the early 1990s. Since the 1960s, it was demonstrated that different genes can be put into cells and that viruses can be used as couriers to deliver these genes. Prof William French Anderson and his colleagues were faced with treating severe combined immunodeficiency (SCID) where patients do not have a functional immune system. They had used a retroviral vector to treat the disease in various animal models and had tested the safety of the viral vector in terminally ill cancer patients. In 1990, Prof Anderson and his colleagues took a pioneering step in the field by using retroviral vectors to treat SCID in two subjects. Their hard work and brilliant rationale paid off and the treatment significantly changed the quality of the lives of these two children”. As of date, more than 2700 clinical trials have been carried out with these modified viruses.
However, there are several considerations and challenges that scientists face when attempting to create the perfect vector. A robust immune response is essential for a vaccine, but in the case of gene therapy, it can make a patient very unwell and/or hamper the efficacy of the therapy. Should the viral genome integrate into the host DNA, the site of integration should be monitored strictly since some viruses can activate oncogenes and/or shut down tumour-suppressor genes. This could create more problems than it solves; no patient would be happy if the therapy cures their diabetes but gives them cancer. Finally, the costs of the treatment has to be kept down. So large amounts of product need to produced using optimal materials. A key issue is that, during production, the recombinant virus may produce a lot of defective particles which might be harmful to the patient.
Where are the opportunities for innovation in gene therapy?
To overcome these challenges, innovative techniques are being used, combining tactics in all of these areas, to increase the efficacy of the therapeutic effect of the vectors.
The immune response can be modified in many ways. The vector can be shielded in molecules so the immune system does not fully recognise it, immune-modulating biomolecules can be expressed to dampen any immune response, or a less immunogenic version of the virus can be used. An opportunity also exists to modify the viral vector itself to extend the duration of the therapy to last for a patient’s lifetime. To reduce the potential toxicity of a therapy due to unexpected insertion in the host genome sites, viruses can be made in clinical grade cell lines and minor alterations that can have major implications in the biotoxicity of the vector, can be introduced to the structure of the viral genome and the therapeutic gene. Finally, the system has to be geared for industrial scale production. Very few people can afford these therapies should they cost an arm and a leg, so the system used for gene therapy has to be easy to manipulate, should produce a lot of good quality vectors and should be easy to access commercially, so that work carried out on them can be brought over to the public who need it, at reasonable costs.
Modified viruses to destroy cancer
Cancer has been a major killer of humanity and the use of recombinant viruses promise hope in banishing this foe. One approach has been to use conditionally replicating viral vectors. As a rule, the replicating potential of a viral vector are limited to the production system. The version that it put in patients is “neutered” and cannot replicate in the patients. In the case of oncolytic viruses, this is different. Cancer is different from other normal tissues. There are different gene products, different temperature and oxidative conditions and different biochemicals. Oncolytic viruses exploit this knowledge and conditionally replicate only in the tumour cells. As they replicate, they destroy the tumour cells in which they grow and move on to the next tumour cell in much greater numbers. At the end of the destruction of the tumour, the virus can no longer replicate in the normal cells and are destroyed by the immune system of the body.
The second approach involves priming the immune system of the body to destroy cancer. The immune system works on the basis of recognition of various molecules some of which stick out of the individual cells like flags. Cancer cells may not wave these “flags” around to hide from the body’s immune system, but they might have totally different “flags”. Chimeric antigen T cell therapy (CAR‑T) system exploits this. The cancer molecules are analysed and a retro/lentiviral vector which carries them is put on the immune cells grown from the human body. Once sufficient numbers of these modified cells are grown up outside the body, they are reintroduced in the body to destroy the cancer. The modified cells will die out at the end of their natural life span.
The current status of viral genetic medicine
The field is moving at a tremendous pace. The recent Ebola epidemic, epidemics by novel and emerging viruses such as Zika, Nipah and Dengue viruses, the presence of rabies, malaria and AIDs all demonstrate the need for novel, effective and cheaper vaccines. The combined annual growth rate (CAGR) for the vaccine field is currently 10.4% and the whole field is estimated to be around $75 billion by 2025. Work with gene therapy vectors have grown exponentially. A number of gene therapy treatments are now available in the clinics with 40 more expected to be approved in the next few years. The CAGR of the field is estimated to be 40.7% with the field expecting to cross the $25 billion mark by 2025.
How can CPI help in this field
At CPI, we have already worked with a number of different universities and companies to assist in the development of various viral vectors and vaccines. We have designed the optimal growth conditions, ideal large-scale purification methods and the best analytical suite (incorporating potency assays) for the analysis of these recombinant viruses. CPI has the drive, knowledge and infrastructure to support the development in this field and it is a very exciting time for me to be at CPI as it helps to bring out these life-altering advanced therapies to the public.
Enjoyed this article? Keep reading more expert insights...
CPI ensures that great inventions gets the best opportunity to become a successfully marketed product or process. We provide industry-relevant expertise and assets, supporting proof of concept and scale up services for the development of your innovative products and processes.